The PhD research conducted as part of the EU-funded SOILPROM Project aims to advance the understanding of the spatial distribution and long-term evolution of pesticide concentrations from the land surface to and within groundwater. The primary focus of this research is to improve the accuracy of bottom boundary conditions in one-dimensional soil models, which depend on groundwater table positions that are often unknown in many European regions.
Objectives and methodology
The research will utilise a coupled soil-groundwater modeling technique to assess the impact of various processes including groundwater flow and solute transport, dilution, and decay, as well as the effects of soil characteristics, groundwater dynamics, meteorological forcing, land-use changes, and pesticide application practices, on the spatiotemporal patterns of pesticide concentrations in the groundwater.
Use-case Zwischenscholle Aquifer – Jülich, Germany
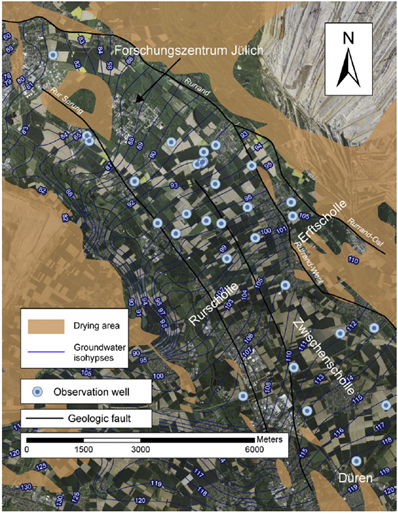
The study area, Zwischenscholle aquifer, is located near Jülich in the Lower Rhine Embayment, Germany (Figure 1). It comprises a mixture of unconfined and semi-confined Quaternary Rhine and Meuse sediments, mainly consisting of sandy and gravelly materials. The base of the aquifer is formed by the water-impermeable Reuver clay. The aquifer thickness varies from a few meters in the southwest to approximately 35 meters in the northeast. Groundwater flows from southeast to northwest with a mean hydraulic gradient between 0.1% and 0.2%. Forests and agriculture are the primary land uses in this area. The typical crop rotation includes sugar beet and winter wheat, while potatoes, oats, and maize are marginally cultivated. Due to the shallow water table depth and intensive agricultural land use, the aquifer’s vulnerability to pesticide contamination is considered very high (Herbst et al., 2005). The Zwischenscholle aquifer is one of the aquifers worldwide contaminated with atrazine, a widely applied herbicide for weed control (Farlin et al., 2013).
Modelling Approach
The study will employ a novel coupling approach between the ParFlow hydrological model (Maxwell et al.,2016; Kuffour et al., 2020) and the HYDRUS-1D soil model (Šimůnek et al., 2016), integrating groundwater table depth data from the DE06 ParFlow/CLM model (Belleflamme et al., 2023). The DE06 ParFlow/CLM setup utilises a Cartesian grid with equidistant lateral spacing, comprising 2,000 × 2,000 grid cells, with a resolution of 0.0055° × 0.0055° (approximately 611 m × 611 m), covering Germany and its neighboring regions (Figure 2). This grid consists of 15 terrain-following vertical model layers, with layer thickness increasing with depth; the uppermost layer extends from the surface to 2 cm, while the lowest layer reaches from 42 m to 60 m below the surface.
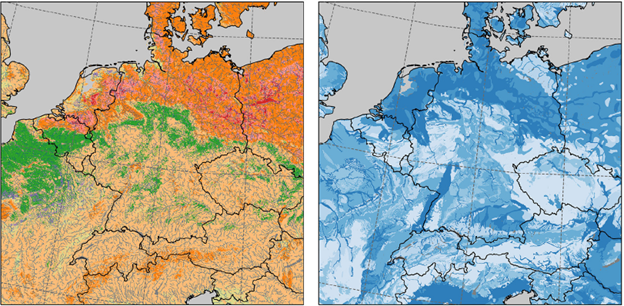
The water table depth outputs from the DE06 ParFlow model (Belleflamme et al., 2024) are utilised to define the bottom boundary conditions for the HYDRUS-1D model. Each HYDRUS-1D model represents a typical soil profile, land cover, depth to groundwater, and weather conditions in the considered zone (Pawlowicz et al., 2024). HYDRUS-1D conducts water flow and solute transport simulations in variably saturated porous media, calculating both the total bottom flux and the solute concentration flux that reaches the groundwater. In turn, the solute flux can be provided as the concentration flux input to the ParFlow model, in conjunction with particle tracking, to simulate solute transport in the saturated zone. This integrated approach enhances boundary condition definitions, leading to more accurate simulations of pollutant leaching.
In this regard, the study area of 21 km² is divided into 504 grid cells, each with dimensions of 200 × 200 m, comprising 14 columns and 36 rows. Considering the highly variable soil hydraulic parameters, surface elevations, water table depths, and atmospheric boundary conditions in the study area, each grid cell is assigned a single HYDRUS-1D profile.
The research aims to incorporate various pesticides and additional contaminants, such as PFAS, based on concerns raised by the regional water authority, project requirements, and the availability of data in the area. Furthermore, we intend to use globally and/or EU-wide available databases, such as ERA5 (Hersbach et al., 2020) or ERA5-Land (Muñoz-Sabater et al., 2021) reanalysis weather products from the ECMWF (European Centre for Medium-Range Weather Forecasts), ASTER global digital elevation model (GDEM) (Abrams et al.,2020; original resolution 1 arc-second)), SoilGrids 2.0 (Poggio et al., 2021) for physical soil properties, and the “International Hydrogeological Map of Europe 1:1,500,000” (IHME1500, Duscher et al., 2015) for the definition of the aquifer types.
Alternative approach
An alternative coupled modeling approach for simulating water flow and contaminant transport in soil and aqufier uses a combination of MODFLOW, MT3DMS, and HYDRUS-1D (Beegum et al., 2018; Beegum et al., 2019). In this setup, MODFLOW (Harbaugh et al., 2000) simulates groundwater flow, HYDRUS-1D models water flow and pollutant transport in the vadose zone, and MT3DMS (Zheng & Wang, 1999) handles solute transport in the saturated zone. Initially, average water table elevations from MODFLOW grids serve as the bottom boundary conditions for HYDRUS-1D profiles. HYDRUS-1D simulates water flow and solute transport in the unsaturated zone for a duration equivalent to MODFLOW time Step 1, calculating total bottom flux and solute concentration flux into groundwater. This bottom flux is used as recharge for the MODFLOW groundwater simulation, which updates the water table level, now assigned as the bottom boundary condition in HYDRUS-1D. Figure 3 shows the conceptual scheme of coupling between HYDRUS-1D and MODFLOW. The solute flux can then be input to MT3DMS for solute transport simulation in the saturated zone.
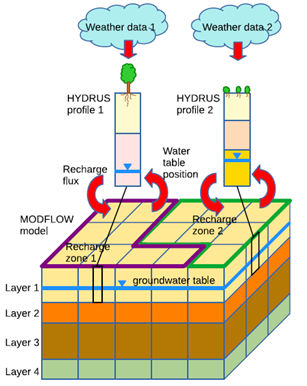
Nonetheless, to build the coupled model using HYDRUS-1D with MODFLOW and MT3DMS, it is essential to have ground-observed water table depth data to define bottom and lateral boundary conditions of the model domain. Unfortunately, such observed data is not available everywhere, making the approach less feasible for areas with limited monitoring data.
ParFlow-HYDRUS-1D Model
The integration between ParFlow and HYDRUS-1D proposed in this research aims to address these limitations by using simulated water table data from the DE06 ParFlow model, along with other publicly available parameters. This approach ensures that the workflow can be transferred to many regions of Europe, particularly sparsely gauged areas, making it highly versatile and adaptable for global applications. This methodology will also be applied in other regions, such as the Rur catchment and other European areas, in collaboration with SOILPROM partners. By building a flexible and adaptable workflow, the research will ensure the scalability and transferability of the modeling framework developed within this study.
The research will then focus on capturing small-scale surface water interactions, such as ditches and creeks, which significantly influence water flow and pollutant transport in both saturated and unsaturated zones. To achieve this, the research will utilise downscaling approaches to accurately capture small-scale water fluxes influenced by localised features such as ditches and creeks. High-resolution models will then be implemented to analyse these interactions in detail. The outcomes of these fine-scale simulations will help parameterise larger-scale models, enabling more accurate predictions of pesticide transport and fate over broader regions.
Implications for sustainable land management
The project’s findings are expected to have implications for sustainable land management strategies, particularly in regions prone to groundwater contamination from pesticide applications. By integrating detailed simulations with advanced modeling techniques, the study aims to support the development of more effective policies and management practices to safeguard water resources.
Through its focus on multi-scale modeling and the integration of advanced hydrological data, this research addresses not only critical scientific challenges but also contributes to the broader objectives of the SOILPROM project, promoting sustainable land and water management practices across Europe.
References
Abrams, M., Crippen, R., & Fujisada, H. (2020). ASTER global digital elevation model (GDEM) and ASTER global water body dataset (ASTWBD). Remote Sensing, 12, 1156. https://doi.org/10.3390/rs12071156
Beegum, S., Šimůnek, J., Szymkiewicz, A., Sudheer, K. P., & Nambi, I. M. (2018). Updating the coupling algorithm between HYDRUS and MODFLOW in the HYDRUS Package for MODFLOW. Vadose Zone Journal, 17, 180034. https://doi.org/10.2136/vzj2018.02.0034
Beegum, S., Šimůnek, J., Szymkiewicz, A., Sudheer, K. P., & Nambi, I. M. (2019). Implementation of solute transport in the vadose zone into the “HYDRUS Package for MODFLOW.” Groundwater, 57, 392–408. https://doi.org/10.1111/gwat.12815
Belleflamme, A., Goergen, K., Wagner, N., Kollet, S., Bathiany, S., El Zohbi, J., Rechid, D., Vanderborght, J., & Vereecken, H. (2023). Hydrological forecasting at impact scale: The integrated ParFlow hydrological model at 0.6 km for climate resilient water resource management over Germany. Frontiers in Water, 5, 1183642. https://doi.org/10.3389/frwa.2023.1183642
Belleflamme, A., Hammoudeh, S., Görgen, K., & Kollet, S. (2024). Experimental FZJ ParFlow DE06 hydrologic forecasts [Data set]. Jülich DATA, V1. https://doi.org/10.26165/JUELICH-DATA/GROHKP
Duscher, K., Günther, A., Richts, A., Clos, P., Philipp, U., & Struckmeier, W. (2015). The GIS layers of the “International Hydrogeological Map of Europe 1:1,500,000” in a vector format. Hydrogeology Journal, 23, 1867–1875. https://doi.org/10.1007/s10040-015-1296-4
Farlin, J., Drouet, L., Gallé, T., Pittois, D., Bayerle, M., Braun, C., Maloszewski, P., Vanderborght, J., Elsner, M., & Kies, A. (2013). Delineating spring recharge areas in a fractured sandstone aquifer (Luxembourg) based on pesticide mass balance. Hydrogeology Journal, 21, 799–812. https://doi.org/10.1007/s10040-013-0964-5
Harbaugh, A. W., Banta, E. R., Hill, M. C., & McDonald, M. G. (2000). MODFLOW-2000, the US Geological Survey modular ground-water model: User guide to modularization concepts and the ground-water flow process. U.S. Geological Survey, 1–92.
Herbst, M., Hardelauf, H., Harms, R., Vanderborght, J., & Vereecken, H. (2005). Pesticide fate at regional scale: Development of an integrated model approach and application. Physics and Chemistry of the Earth, Parts A/B/C, 30, 542–549. https://doi.org/10.1016/j.pce.2005.07.009
Hersbach, H., Bell, B., Berrisford, P., Hirahara, S., Horányi, A., Muñoz-Sabater, J., et al. (2020). The ERA5 global reanalysis. Quarterly Journal of the Royal Meteorological Society, 146(730), 1999–2049. https://doi.org/10.1002/qj.3803
Kuffour, B. N. O., Engdahl, N. B., Woodward, C. S., Condon, L. E., Kollet, S., & Maxwell, R. M. (2020). Simulating coupled surface-subsurface flows with ParFlow v3.5.0: Capabilities, applications, and ongoing development of an open-source, massively parallel, integrated hydrologic model. Geoscientific Model Development, 13, 1373–1397. https://doi.org/10.5194/gmd-13-1373-2020
Maxwell, R. M., Kollet, S. J., Smith, S. G., Woodward, C. S., Falgout, R. D., Ferguson, I. M., et al. (2016). ParFlow User’s Manual. Integrated GroundWater Modeling Center Report GWMI, 167p.
Muñoz-Sabater, J., Dutra, E., Agustí-Panareda, A., Albergel, C., Arduini, G., Balsamo, G., et al. (2021). ERA5-Land: A state-of-the-art global reanalysis dataset for land applications. Earth System Science Data, 13, 4349–4383. https://doi.org/10.5194/essd-13-4349-2021
Pawlowicz, M., Balis, B., Szymkiewicz, A., Šimůnek, J., Gumuła-Kawęcka, A., & Jaworska-Szulc, B. (2024). HMSE: A tool for coupling MODFLOW and HYDRUS-1D computer programs. SoftwareX, 26, 101680. https://doi.org/10.1016/j.softx.2024.101680
Poggio, L., de Sousa, L. M., Batjes, N. H., Heuvelink, G. B. M., Kempen, B., Ribeiro, E., & Rossiter, D. (2021). SoilGrids 2.0: Producing soil information for the globe with quantified spatial uncertainty. SOIL, 7, 217–240. https://doi.org/10.5194/soil-7-217-2021
Vonberg, D., Vanderborght, J., Cremer, N., Putz, T., Herbst, M., & Vereecken, H. (2014). 20 years of long-term atrazine monitoring in a shallow aquifer in western Germany. Water Research, 50, 294–306. https://doi.org/10.1016/j.watres.2013.10.032
Zheng, C., & Wang, P. P. (1999). MT3DMS: A Modular Three-Dimensional Multispecies Transport Model for Simulation of Advection, Dispersion, and Chemical Reactions of Contaminants in Groundwater Systems: Documentation and User’s Guide. University of Alabama: Tuscaloosa, AL, USA.
Šimůnek, J., van Genuchten, M. T., & Šejna, M. (2016). Recent developments and applications of the HYDRUS computer software packages. Vadose Zone Journal, 15. https://doi.org/10.2136/vzj2016.04.0033
Authors
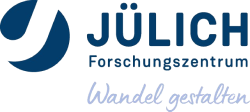
Ali Sadrzadeh is a doctoral researcher at the Agrosphere Institute (IBG-3) of Forschungszentrum Jülich, Germany. He obtained an Erasmus Mundus Joint Master’s Degree in Groundwater and Global Change – Impacts and Adaptation (GroundwatCH) from Instituto Superior Técnico (Portugal), IHE Delft (Netherlands), and TU Dresden (Germany). He is currently contributing to the SOILPROM project, where he focuses on simulating water flow and pesticide transport in unsaturated and saturated zones using a coupled soil-groundwater modeling approach.
Prof. Dr. Jan Vanderborght is the Director of the Agrosphere Institute (IBG-3) at Forschungszentrum Jülich, Germany. He also serves as a professor at KU Leuven’s Faculty of Bioscience Engineering. His research focuses on solute transport in soils, pesticide fate, hydrogeophysics, soil evaporation, and plant uptake of water, nutrients, and pesticides. In recognition of his contributions, he was named a Fellow of the Soil Science Society of America (SSSA) in 2024.
Dr. Lutz Weihermüller is a senior researcher specializing in soil physics at the Agrosphere Institute (IBG-3) at Forschungszentrum Jülich, Germany. He is responsible for the soil physical laboratories at IBG-3, focusing on determining soil hydraulic characteristics. His research encompasses soil physics, numerical modeling, hydrogeophysics, carbon turnover, and the fate of organic pollutants. Dr. Weihermüller is also the coordinator of the International Soil Modelling Consortium (ISMC).